MicroRNAs, snippets of RNA that were first recognized only 2 decades ago, are turning out to be important players in a wide variety of biological processes. They have been implicated, for example, in embryogenesis, cholesterol metabolism, learning and memory, and cancer. Now, two studies by NIDA-funded scientists present evidence that microRNAs also regulate genes involved in drug addiction. Their findings offer a new direction for the development of anti-addiction therapies.
"We have been thinking for a long time that microRNAs might be involved in drug addiction because addiction is such a complex disorder and microRNAs are known to influence multiple circuits in the brain," says Dr. Paul Kenny of The Scripps Research Institute in Jupiter, Florida. "We have spent quite a few years trying to figure out what they might do."
In one recent study, Dr. Kenny and colleagues found that rats that generate high levels of one particular microRNA in the brain following extended cocaine exposure are less likely to exhibit addiction-like behaviors. The researchers also traced some of the molecular pathways through which this microRNA exerts its effects.
Master Regulators of Gene Expression
MicroRNAs, like the better known messenger RNAs, are strings of nucleotides arranged in specific sequences. As their name suggests, microRNAs are far shorter than messenger RNAs, averaging only about 22 nucleotides as opposed to hundreds or thousands.
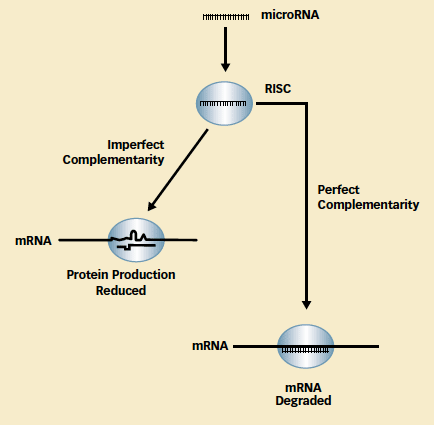
Functionally, microRNAs interact with messenger RNAs to regulate protein production. The interaction is push-pull in nature: Messenger RNAs execute an essential production step by conveying protein-building instructions from genes to the protein- synthesizing machinery of cells, and microRNAs prevent them from doing so. The relative abundance of the two types of RNAs thus determines the amount of protein produced.
There are approximately 1,000 different microRNAs in human cells, and each attaches to a complementary RNA segment—its "ID code"—present in a messenger RNA (see illustration). The microRNA binds to the messenger RNA as part of a complex with several proteins, called an RNA-induced silencing complex (RISC), that either destroys the messenger RNA or prevents it from making protein.
Because many different messenger RNAs may contain the same ID code, each microRNA may affect the expression of many genes. Researchers suspect that each microRNA can bind to hundreds or thousands of messenger RNA targets, while each messenger RNA can have binding sites for dozens or even hundreds of different microRNAs.
Dr. Kenny's new work is the first demonstrating a possible role for microRNAs in regulating genes involved in drug addiction. Researchers have shown that addictive drugs affect the expression of many genes in the brain and have implicated some of these alterations in addictive behaviors such as drug craving and compulsive drug use.
Protection Against Addiction
In initial experiments with rats, Dr. Kenny and colleagues identified microRNAs that increase in abundance in the brain's dorsal striatum following extensive exposure to cocaine. One of these, miR-212, appeared to inhibit avid drug-seeking in rodents.
The researchers compared microRNA levels in brain tissue from two groups of rats. One group had freely self-administered cocaine for a week in daily 6-hour sessions; the other, in daily 1-hour sessions. Previous research has shown that rats exhibit addiction-like behaviors—in particular, accelerated, uncontrolled cocaine intake—following the 6-hour regimen but not the 1-hour regimen. Although both groups showed the effects of cocaine exposure per se on brain chemistry, only the 6-hour animals showed changes specifically related to the addictive process. Among the differences, the researchers found twice as much miR-212 in the dorsal striatum of the 6-hour animals as compared with that in 1-hour animals (see figure). Another microRNA, miR-132, showed a similar pattern of expression, but its role in regulating drug addiction has yet to be analyzed.
In further work, Dr. Kenny and colleagues investigated how increases in miR-212 affect the addictive process. Surprisingly, they found that such increases actually mitigate addictive behaviors.
In this experiment, the researchers introduced a benign virus containing extra copies of the miR-212 gene into the dorsal striatum of rats. This maneuver exaggerated the cocaine-induced increase in miR-212 and demonstrated its behavioral impact. Whereas normal rats steadily increased their cocaine intake throughout a week on the 6-hour regimen, the gene-enhanced animals reduced their intake. In addition, normal animals exhibit another behavioral signature of addiction, a marked preference for higher doses of the drug, but animals with extra miR-212 did not. Conversely, when the researchers blocked miR-212 function, the extended-access animals consumed the drug in increasing amounts and preferred higher doses to lower ones.
The researchers concluded that the brain's increased production of miR-212 following prolonged cocaine exposure may represent a self-protective response against drug-induced changes that underlie addiction. "Individuals who cannot mount a response to cocaine exposure by producing more miR-212 are probably more vulnerable to addiction," says Dr. Kenny.
Pathways
Dr. Kenny and colleagues uncovered three mechanisms by which miR-212 preserves animals' control over drug intake. The microRNA weakens animals' motivation to self-administer the drug by:
- Promoting increased activity of a transcription factor called CREB (cAMP response element binding protein) that dampens sensitivity to cocaine's rewarding effects;
- Inhibiting production of a molecule called BDNF (brain-derived neurotrophic factor) that enhances sensitivity to cocaine's rewarding effects;
- Inhibiting production of a molecule called methyl CpG binding protein 2 (MeCP2) that is closely linked to BDNF and also intensifies sensitivity to cocaine reward.
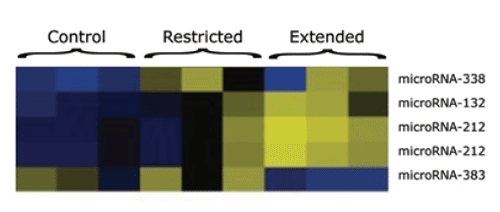
Further work by the Scripps team placed miR-212 within a delicately balanced network of epigenetic feedback and feed-forward loops in the dorsal striatum. CREB is pivotal in the network because its effects are bidirectional. Overall, it reduces sensitivity to cocaine reward, but it also promotes production of BDNF, which has the opposite effect. CREB tempers this second effect, however, by also promoting miR-212, which reduces production of BDNF and competes with MeCP2. Ultimately, the sum of the direct and indirect, reciprocal and opposed relationships among all of these molecules shapes an individual's vulnerability to cocaine's motivational effects.
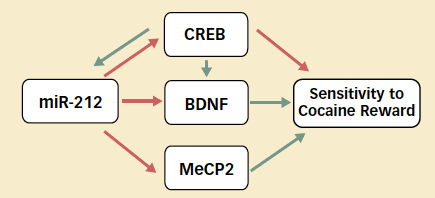
Dr. Kenny calls particular attention to MeCP2 in this network. Mutations in this protein have been implicated by other researchers in Rett syndrome, a neurodevelopmental disorder that occurs mainly in girls and is related to autism. Like addicted individuals, people with Rett syndrome have abnormal function in the dorsal striatum and behave compulsively.
The Scripps researchers' finding, that MeCP2 is implicated in uncontrolled drug-taking, provides support for an idea that had been suggested but never confirmed: that addiction and neurodevelopmental processes may sometimes share pathways.
More MicroRNAs Linked to Addiction
Mice whose neurons lack a protein essential for the generation or function of certain microRNAs consume less cocaine than normal mice, according to a study by the team of Dr. Paul Kenny of The Scripps Research Institute in Jupiter, Florida, in collaboration with NIDA-supported investigator Dr. Paul Greengard of The Rockefeller University. The results suggest that some microRNAs may stimulate brain mechanisms that contribute to drug addiction.
The investigators blocked the expression of the gene Argonaute 2, which produces the protein (Ago2) that is essential for many microRNAs to function. The Ago2-deficient mice showed reduced motivation to self-administer cocaine.
The researchers examined a group of brain neurons that express dopamine 2 receptors (D2Rs). These neurons are of particular interest because the neurotransmitter dopamine is implicated in drug abuse and addiction, and animals with fewer available D2Rs have been shown to be especially responsive to cocaine's reinforcing effects.
In their experiment, the researchers identified 23 microRNAs that have reduced abundance or function in D2R-expressing neurons of the Ago2-deficient animals. From the nucleotide sequences, the researchers predict that those microRNAs will affect the activity or production of several compounds, including CREB and BDNF, which are already known to play roles in cocaine addiction. Future studies may identify the contribution of each of the microRNAs to various facets of addictive behavior.
This study suggests that unlike miR-212, which tends to reduce an animal's cocaine intake, some microRNAs promote cocaine intake. "There is likely to be tremendous interplay between all the microRNAs in the brain," Dr. Kenny says. "Together, they coordinate responses to cocaine and vulnerability to addiction."
Source
Schaefer, A., et al. Argonaute 2 in dopamine 2 receptor-expressing neurons regulates cocaine addiction. Journal of Experimental Medicine207(9):1843-1851, 2010. (Full Text (PDF, 4.9MB))
Opening the Door to New Therapies
A model of addiction is emerging from these two studies by Dr. Kenny and colleagues. It suggests that miR-212 serves as a key regulator of CREB and BDNF and other molecules in the striatum, thus affecting cocaine intake. Other microRNAs may play similar roles, resulting in intricate networks of regulation of the brain's reward pathways.
Dr. Kenny and colleagues have already discovered that levels of other microRNAs, such as miR-132 (see figure - Spot the Difference - above), also increase in the dorsal striatum of rats given extended access to cocaine. However, some of these other microRNAs, unlike miR-212, may promote addiction rather than protect against it (see box).
"These studies provide the first strong evidence that microRNAs play a role in drug abuse," says Dr. John Satterlee of NIDA's Division of Basic Neuroscience and Behavioral Research.
One exciting aspect of this work is that it may be possible to develop treatments for drug addiction or other disorders by replicating the actions of a particular microRNA. Therapies based on microRNAs are already being tested in cancer, with some currently in randomized controlled multicenter trials on large patient groups. "If we tweak these therapies in just the right way, they might be very beneficial for treating drug addiction," says Dr. Kenny, adding that he is cautiously optimistic about the prospects.
There are, however, many challenges to developing microRNA therapies that treat addiction. Any therapy targeted to the brain, for example, has to make its way across the barrier of tightly fitting endothelial cells that line blood vessels, preventing many substances from passing from the blood into the brain. This barrier evolved to protect the brain from poisons, but it also keeps many medications out. In addition, because one microRNA may regulate thousands of genes, harmful side effects might result from turning on or off the production of a microRNA.
Despite these caveats, Dr. Kenny's work opens the door to a new therapeutic approach, according to Dr. Satterlee. "MicroRNAs and the pathways they regulate provide a huge number of potential drug targets that we did not know about," he says.
Sources
Hollander, J.A., et al. Striatal microRNA controls cocaine intake through CREB signaling. Nature 466(7303):197-202, 2010. (Full Text (PDF, 4.1MB))
Im, H.I., Hollander, J.A., Bali, P., and Kenny, P.J. MeCP2 controls BDNF expression and cocaine intake through homeostatic interactions with microRNA-212. Nature Neuroscience 13(9):1120-1127, 2010. (Full Text (PDF, 2.4MB))