Researchers have shut down laboratory rats’ compulsive cocaine seeking by stimulating an area of the animals’ prefrontal cortex (PFC). The finding raises the possibility that stimulating neurons in this brain area may weaken or break cocaine’s grip on the behavior of people who are addicted to the drug.
Dr. Billy Chen, Dr. Antonello Bonci, and colleagues at the NIDA Intramural Research Program (IRP) in Baltimore, Maryland, conducted experiments to better understand the significance of reduced PFC activity in drug abuse and addiction. Their findings demonstrate that cocaine dampens neuronal excitability in the rat PFC, and suggest that cocaine use becomes compulsive when PFC hypoactivity crosses a threshold of severity. They also found that, at least in rats, redressing the falloff in PFC activity can curb the compulsion.
The PFC and Compulsion
Only about 1 in 5 people who try cocaine progress to compulsive use and addiction. Dr. Chen and his colleagues hypothesized that what distinguishes this 20 percent from their more resistant peers is greater impairment of PFC function.
“The idea makes sense that once you lose function in this area, you have less control over your behavior,” says Dr. Chen, now at Isis Pharmaceuticals in Carlsbad, California. The PFC is largely responsible for self-control—that is, the power to look before leaping, anticipate negative consequences, and refrain from actions likely to yield negative results. Moreover, brain imaging studies have demonstrated that people with substance use disorders have reduced activity in the PFC, and have linked this abnormality to these individuals’ poor decision-making.
Unmasking Compulsiveness in Rats
Rats, like people, vary in their susceptibility to compulsive cocaine use. Dr. Chen and colleagues thus were able to compare PFC function in animals that developed compulsive drug seeking and in animals that did not.
The researchers trained rats to press two levers in succession to receive infusions of cocaine. Pressing the first (“seek”) lever gave access to the second (“take”) lever, the pressing of which delivered the drug. Animals could earn up to 30 infusions of cocaine in each daily training session.
After the rats had self-administered cocaine in this way for about 2 months, the researchers added a wrinkle to sort compulsive from noncompulsive drug seekers: A mild electric shock to the foot was delivered in 30 percent of the trials. After 4 days of this regimen, 30 percent of the rats continued to seek cocaine despite the shocks, an indicator of compulsive drug seeking. The other 70 percent of rats stopped seeking cocaine almost immediately. They scooted quickly away from the lever after the first shock; some even retreated to the opposite corner of the cage for the rest of the 6-hour session.
Dr. Chen and colleagues then compared neuronal function in the PFC between the two groups of rats, and with a group of rats that had not been exposed to the drug.
Faulty Neuron Firing Removes Inhibitions
The researchers specifically examined neurons in the prelimbic area of the rats’ PFC. This area corresponds to the dorsal lateral prefrontal cortex in the human PFC, which plays a central role in decision-making: It sends signals to other brain areas to inhibit them from initiating actions whose likely downsides exceed their likely benefits.
The compulsive rats’ prelimbic neurons were significantly less excitable than those of the noncompulsive rats: The researchers needed to apply almost twice as much external current to get them to generate an action potential (see Figure 1). The compulsive rats’ neurons also showed impaired repetitive firing, another indication of low neuron responsiveness.
“This probably means that even if a lot of information is coming into the PFC, the brain of a compulsive rat is unable to transmit this information to its downstream targets,” says Dr. Chen. “It is tempting to speculate that in a compulsive rat, the PFC is unable to relay the information that pressing the seek lever is associated with a foot shock, rendering the animal unable to stop itself.”
Both groups of cocaine-exposed rats had less excitable PFC neurons than the unexposed control animals. This finding sheds light on a vexing chicken-and-egg question raised by the observation that the PFC is hypoactive in human cocaine users: Which comes first, the deficient PFC or the drug use? The new evidence strongly suggests that whatever an individual’s level of PFC function may be prior to initiation of cocaine use, long-term drug use worsens it.
Cocaine may inhibit neuronal firing in various ways, says Dr. Chen. For example, the drug may alter gene expression or disrupt neurotransmitters such as dopamine or serotonin.
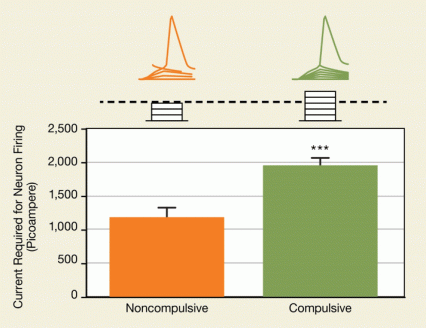
- Text description of Figure 1
-
The figure shows differences in firing of action potentials of neurons in the prelimbic cortex between rats seeking cocaine noncompulsively or compulsively. The top of the upper panel shows traces of action potentials (shown in orange color for noncompulsive and in green for compulsive rats) triggered in response to externally applied 2-ms current pulses indicated as stacks of rectangles below each of the peaks; the neurons in rats seeking cocaine compulsively required a greater number of pulses to exceed the threshold for generation of an action potential. The y-axis of the graph in the lower panel shows the amount of external current required (in picoamperes) to generate an action potential, and the x-axis shows the two different groups of rats (noncompulsive and compulsive, whose data are shown as orange and green bars, respectively) in which the action potential responses were compared. The difference in the heights of the two bars show that prelimbic neurons in the compulsive cocaine seekers required almost twice as many current injections as those in the noncompulsive cocaine seekers to generate an action potential, and this difference was statistically significant (indicated by the three asterisks at the top of the bar for the compulsive cocaine seekers, denoting a p value of less than 0.001).
Across the Threshold—and Back
Dr. Chen and colleagues drew upon a new investigative technique to further solidify and extend their findings. In these experiments, inhibiting rats’ prelimbic neurons converted them from noncompulsive to compulsive cocaine seekers, while stimulating those neurons had the opposite effect.
The investigative technique, optogenetics, uses light-sensitive proteins to control the firing of individual neurons or small groups of neurons in live animals. The IRP researchers induced the neurons in the prelimbic cortex of experimental rats to manufacture a light-sensitive protein called halorhodopsin and express (install) it in the neuronal membrane. When activated by light of a particular wavelength, halorhodopsin draws negative ions into the cell, dampening neuronal activity.
The results were dramatic. When the researchers used an implanted fiberoptic filament to shine the light into the prelimbic cortex, rats that had previously not compulsively sought cocaine began to do so. Whereas before they had avoided the electrified “seek” lever, they no longer did so.
The researchers then reversed the experiment. “We thought, ‘Let’s make the quiet neurons in compulsive rats fire better and see what happens,’ ” Dr. Chen says. If decreasing neuron activity beyond a threshold triggers compulsive drug seeking, then raising activity above that threshold might restore self-control.
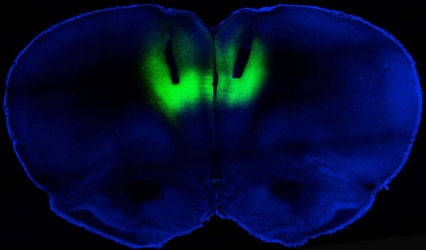
- Text description of Figure 2
-
The figure shows a cross-sectional brain slice of a rat genetically engineered to express the channelrhodopsin-2 protein, which functions as an ion channel protein that opens in response to stimulation by blue light. Channelrhodopsin-2 was fused to a fluorescent protein (eYFP) to visualize its location. Both proteins were expressed in the membranes of neurons via injection of a virus into the rat’s prelimbic cortex. The image shows the location of the channelrhodopsin-2–eYFP protein fusion in the prelimbic cortex, visualized by the green glow in response to illumination by blue light; in the experiments with live rats, optical fibers were used for targeted stimulation of channelrhodopsin-2 while rats were offered cocaine.
To evaluate this possibility, the IRP team again turned to optogenetics. This time, they caused rats’ prelimbic neurons to express channelrhodopsin-2 (ChR-2) (see Figure 2). Exposing ChR-2-expressing neurons to light of an appropriate wavelength increases the inward flow of positive ions and triggers neuronal firing. When the researchers switched the light on simultaneously with the presentation of the cocaine “seek” lever that was wired to give foot shocks, the rats significantly decreased their compulsive cocaine-seeking activity.
“I was very surprised that when we boosted neuronal activity in prelimbic neurons, the rats’ behavior was very quickly altered,” says Dr. Chen. In fact, their compulsive behavior dropped off as soon as the light came on at the start of the session. The animals doubled their waiting time between consecutive presses of the lever, and consumed only half as much cocaine, compared with their intake without optogenetic stimulation (see Figure 3).
“An important future study will be to figure out what the differences are that lead animals to be susceptible to prelimbic hypoactivity and compulsive behavior when exposed to cocaine,” says Dr. Chen. Meanwhile, he says, the current work has expanded our fundamental understanding of the brain’s ability to regulate inhibitory control: “How do you stop yourself from doing things you don’t want to do?”
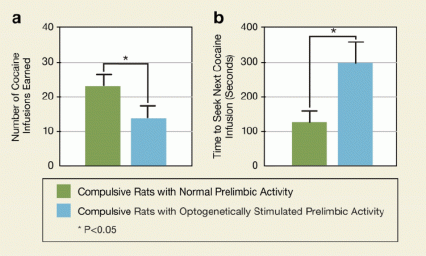
- Text description of Figure 3
-
The y-axis of the graph on the left (a) shows the number of cocaine infusions earned and the x-axis shows two groups of rats compulsively seeking cocaine that had either normal prelimbic neurons (green bar) or prelimbic neurons that were optogenetically stimulated to increase firing (blue bar). The difference in the heights of the bars between the two groups indicates that compulsive rats whose prelimbic cortex was optogenetically stimulated earned fewer cocaine infusions than the compulsive rats with normal prelimbic activity, and this difference was statistically significant (indicated by asterisk, denoting a p value of less than 0.05). The y-axis of the graph on the right (b) shows the amount of time (in seconds) it took before a rat sought the next cocaine injection for the same two groups of rats as shown in the graph on the left (a). The difference in bar height between the two groups indicates that compulsive rats whose prelimbic cortex was optogenetically stimulated waited longer to receive a new cocaine infusion than the compulsive rats with normal prelimbic activity, and this difference was statistically significant (indicated by asterisk, denoting a p value of less than 0.05).
On the Horizon: Clinical Trials
“Studies of people with substance use disorders suggest that their deficits in inhibitory control may also result from a hypoactive frontal cortex,” says Dr. Chen. If that is so, then finding a way to repair this PFC defect could lead to therapies for those with substance use disorders or, possibly, for those who struggle with other compulsive, destructive behaviors.
Dr. Chen and colleagues are already planning a clinical trial to test this possibility using transcranial magnetic stimulation (TMS) to facilitate abstinence from cocaine. TMS employs external magnets to stimulate targeted areas of the brain through the skin and skull. The technology is currently being tested as a potential therapy for a variety of conditions; it has already shown some promise for reducing cravings in tobacco addiction.
Source:
Chen, B.T.; Yau, H.J.; Hatch, C.; Kusumoto-Yoshida, I.; Cho, S.L.; Hopf, F.W.; Bonci, A. Rescuing cocaine-induced prefrontal cortex hypoactivity prevents compulsive cocaine seeking. Nature 496(7445): 359-362, 2013. Abstract